Common-spectrum process versus cross-correlation for gravitational-wave searches using pulsar timing arrays, by Joseph D. Romano, Jeffrey S. Hazboun, Xavier Siemens, and Anne M. Archibald
Pulsar timing arrays are trying to detect gravitational waves with periods of years by sensing their effect on the arrival time of pulses from pulsars all over the sky. A gravitational wave passing over the Earth compresses space in some directions and expands it in others, and this should produce a correlated pattern of delays between multiple pulsars. At these frequencies, the signal we expect to detect is a mixture of gravitational waves from all the supermassive black hole binaries in the Universe; this should look like random noise with a power-law spectrum, strongest at the lowest frequencies. Recently, NANOGrav detected hints of such a spectrum. It detected these hints not in the cross-correlations between pulsars, but as a common spectrum of noise in the autocorrelations of individual pulsars. By contrast, LIGO’s attempt to detect a stochastic gravitational-wave background is expected to find evidence in the cross-correlations first. (And of course we will find cross-correlations much more convincing evidence for a gravitational-wave origin than auto-correlations – there are many fewer alternative sources of cross-correlated noise.) So why did NANOGrav find evidence first in the autocorrelations? This paper uses a simple toy model to explain why.
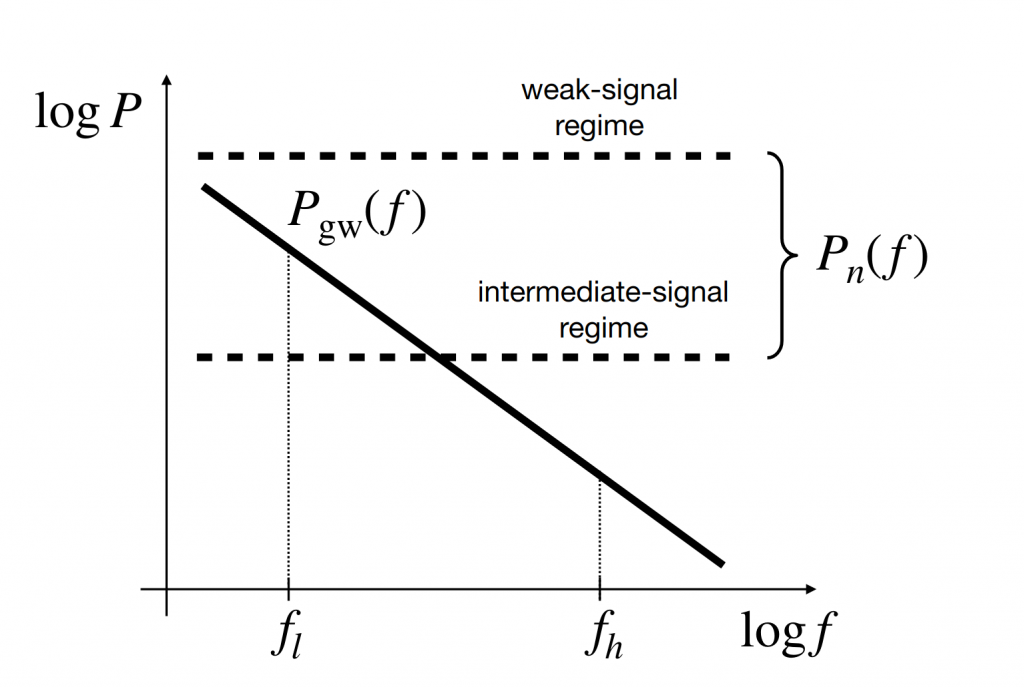