Magnetohydrodynamics (MHD)/Dynamos
Convection and Dynamos in Planetary Interiors
Cooper, R.G. (supervised by Guervilly, C. & Bushby, P.)(2018)
PhD Project
MHD-Days-GdRI-2018 – my most in depth talk on my research at the MHD Days & GdRI Dynamo Meeting in Dresden.
STFC-Southampton-2018 – a brief talk at the STFC Advanced Summer School 2018 in Southampton on my work so far.
Many planets have a magnetic field that is generated by a dynamo process from the motion of conducting fluids inside planetary interiors, such as in the Earth. In magnetohydrodynamics (MHD), convection in a rotating, electrically conducting fluid acts to maintain a magnetic field. In a planet this fluid is driven by gradual cooling in the interior of the planet. This can happen if the convection is able to produce a magnetic field strong enough to alter the structure of the convective flows. Interestingly, some planets such as Mars do not presently have a magnetic field, but show evidence of having one in the past. The Mars Global Suveryor revealed that the rocks on the surface have strong remnant magnetisation despite there being no global magnetic field (Acuña et al. (1999)). Studies observe that the cessation of the Martian dynamo occured rapidly (Lillis et al. (2008)). One possible cause of this sudden termination is subcritical dynamo action. That is, dynamo action for convective forcing below the threshold necessary for convective motions to occur in the absence of magnetic fields.
In the subcritical regime, the energy required to sustain the dynamo is far less than required to initiate the dynamo devoid of the strong magnetic field. Since the energy in the subcritical regime is lower than that required to generate the dynamo, if the Martian dynamo was ceased then it may not be possible for it to restart. This is the scenario that I will explore in my thesis.
Localized States in Rotating Convection at Low Prandtl Number
Cooper, R.G. (supervised by Guervilly, C. & Bushby, P.)(2018)
PhD Sub-project
PG-Applied-Conference-2018 – recent talk given at the Newcastle University Postgraduate Applied Maths Conference 2018.
UKMHD-2018 – poster presented at UK MHD 2018 at University of St Andrews.
As the first sub-project of my PhD we are interested in the formation of large-scale patterns in rapidly-rotating convection, such as the convective nests observed in 3D spherical convection (Grote & Busse, 2001; Brown et al., 2008), which might explain why some planets such as Mercury generate weak dipolar fields (Heimpel et al., 2005). We consider the most basic setup where localized convective structures are observed (Beaume et al., 2013) and study the motion of a layer of fluid in a rotating box, uniformly heated from below. We also consider the system at low Prandtl numbers (Pr=0.025), relevant to planetary cores.
Quantum Fluids
Publication: Knot Spectrum of Turbulence
Cooper, R.G., Mezgarnezhad, M., Baggaley, A.W. and Barenghi, C.F. (2019), Knot spectrum of turbulence, Scientific Reports, 9, 10545
https://www.nature.com/articles/s41598-019-47103-w
Streamlines, vortex lines and magnetic flux tubes in turbulent fluids and plasmas display a great amount of coiling, twisting and linking, raising the question as to whether their topological complexity (continually created and destroyed by reconnections) can be quantified. In superfluid helium, the discrete (quantized) nature of vorticity can be exploited to associate to each vortex loop a knot invariant called the Alexander polynomial whose degree characterizes the topology of that vortex loop. By numerically simulating the dynamics of a tangle of quantum vortex lines, we find that this quantum turbulence always contains vortex knots of very large degree which keep forming, vanishing and reforming, creating a distribution of topologies which we quantify in terms of a knot spectrum and its scaling law. We also find results analogous to those in the wider literature, demonstrating that the knotting probability of the vortex tangle grows with the vortex length, as for macromolecules, and saturates above a characteristic length, as found for tumbled strings.
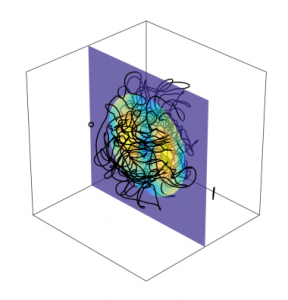
Three-dimensional snapshot of the vortex tangle superimposed onto the magnitude of the driving flow.
Publication: Helicity and Topology of a Small Region of Quantum Vorticity
Mezgarnezhad, M., Cooper, R.G., Baggaley, A.W. and Barenghi, C.F. (2018), Helicity and topology of a small region of quantum vorticity, Fluid Dynamics Research, 50(1), 011403
http://iopscience.iop.org/article/10.1088/1873-7005/aa694f
We numerically study the evolution of a small turbulent region of quantised vorticity in superfluid helium, a regime which can be realised in the laboratory. We show that the turbulence achieves a fluctuating steady-state in terms of dynamics (energy), geometry (length, writhing) and topology (linking). After defining the knot spectrum, we show that, at any instant, the turbulence consists of many unknots and few large loops of great geometrical and topological complexity.
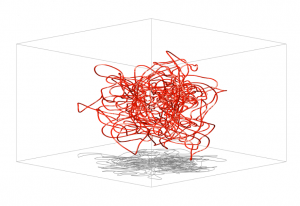
A vortex tangle, we find one vortex loop of extremely high topological complexity and many of low or trivial complexity.
Topology of Superfluid Turbulence: Computing the Alexander Polynomial
Cooper, R.G. (supervised by Barenghi, C.F.) (2017)
Newcastle University MMath 2017
MMath-Dissertation – masters dissertation.
MMath-Poster – masters poster.
We seek to determine the topological complexity of a small patch of turbulence in superfluid helium away from solid or periodic boundaries. Since superfluids have quantised vorticity we observe the formation of tangled filamentary structures which evolve over time. With the aid of the knot invariant known as the Alexander polynomial, we show that at any time step our tangle consists of mostly unknots and vortex knots of low complexity but that there always exist some loops of high geometrical and topological complexity. We then go on to compare the topological complexity with the geometry and dynamics of the system finding a clear increase in complexity with relation to geometrical properties such as length and writhe.